Many Faces, and Phases, of Steel in Cars
For all the talk of reinvention in the auto industry, of car companies using high-tech materials to make lighter and stronger vehicles that are safer and more fuel-efficient, the mainstay of the mass-produced automobile is the same as it has always been: steel. The modern car still contains more of it than anything else, about 60 percent by weight.
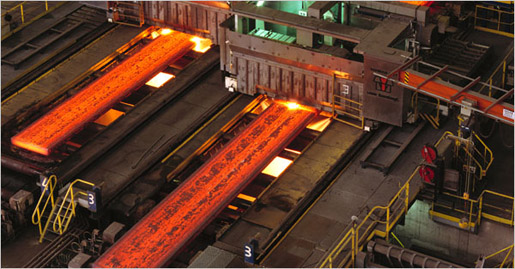
But automotive steel has changed quite a bit since the first Model T rolled off the assembly line. Metallurgists and manufacturers have learned to manipulate steel’s microstructure through precise control of processing to create sheet steels of increasing strength. Prompted by crash-worthiness requirements and the need to make cars lighter to improve gas mileage, automakers are replacing conventional steels with advanced high-strength ones.
Where once a single grade of steel might have sufficed, the typical “body in white,” as automakers call a car’s basic skeleton, might now be a patchwork of a dozen or more steels of different types and strengths, tailored through computer modeling to handle the stress and strain of normal driving — and of severe crashes.
“The day of the mild steel part at the Ford Motor Company is dead,” said Ford’s chief safety engineer, Steve Kozak. “The majority of steels we’re using now are high strength or ultra high strength.”
The advanced steels go by names like D.P. (for dual phase) and TRIP (for transformation-induced plasticity). The strongest ones are used in parts like door beams, where the aim is to stop a foreign object (like another car’s bumper) from entering the passenger compartment, and windshield pillars, where the goal is to prevent the roof from flattening like a pancake in a rollover.
In the front and rear of the vehicle, where there is more room to absorb the energy of a collision, steels that deform more easily, and get stronger as they do, might be used. Even body panels, which are usually made from milder steel, are bake hardenable, getting stronger as they are heated during paint curing to resist denting better.
North American carmakers draw a lot of criticism for overall design and reliability of their cars, but they generally receive high marks for their use of advanced steels. “I’d say North America doesn’t have to take a back seat to anyone,” said Richard A. Schultz of Ducker Worldwide, a consulting firm that tracks materials use by automakers.
The steel industry, Mr. Schultz said, has worked to develop new products, to keep steel in cars and to fend off the increased use of aluminum and other materials. They have been developing higher-strength products for decades, beginning with high-strength low-alloy, or H.S.L.A., steels. But most were costly to produce and difficult to make into parts. “They were looked at as more for aerospace applications,” Mr. Schultz said.
Only in recent years have researchers and manufacturers figured out how to make economical high-strength steels that are pliant enough to be stamped and formed and that can be welded or otherwise joined to other parts in the complex auto assembly process, where time is money.
“At the end of the day we have to manufacture everything that’s there,” said James G. Schroth, lab group manager in the Materials and Processes Lab of General Motors’ Research and Development Center. “The real changes in technology have been the higher-strength materials we can still make into parts.”
Using higher-strength steel, a part can be downgauged — made from thinner stock — to help improve fuel economy. But some parts, notably exterior panels, are already so thin — less than a millimeter, or one twenty-fifth of an inch, in many cases — that stiffness becomes an issue. “You reach a thickness where you can’t go even thinner,” Mr. Schroth said.
Like other steels, high-strength varieties begin as molten iron produced from ore and coke in a blast furnace. “Despite literally hundreds of years of development of the process, it’s still the best and most economical way of making high-purity iron,” said Blake K. Zuidema, director of automotive product applications for the steelmaker ArcelorMittal.
The molten iron, usually with scrap added, is made into steel in a basic oxygen furnace, in which oxygen is blown into the melt (“basic” in the name refers to the alkaline materials used to line the furnace). The gas oxidizes the iron and removes some impurities and most of the carbon. (High-carbon steel is very hard but difficult to work.)
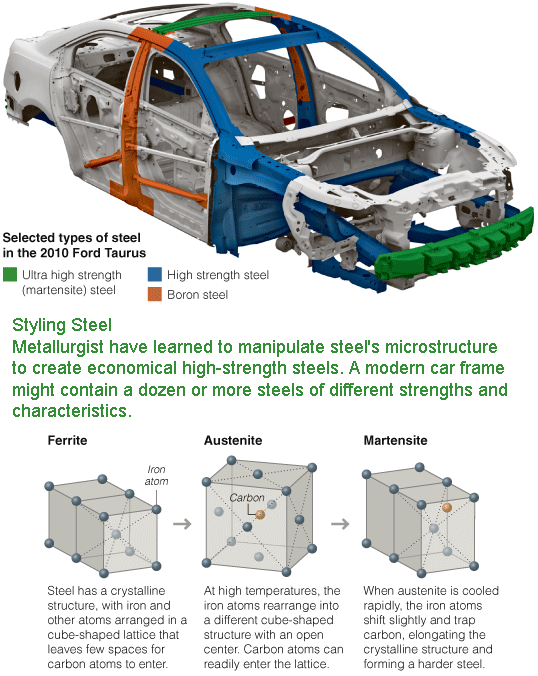
For most automotive purposes, the steel is cast into a slab. At this point, Dr. Zuidema said, “you have a product that is not too much different than conventional steels,” except for the addition of small amounts of other metals — alloys.
Some of the strength of steel is gained through alloying, said David K. Matlock, a metallurgist at the Colorado School of Mines and director of the Advanced Steel Processing and Products Research Center, which is supported by auto and steel companies.
Small amounts of metals like niobium or vanadium help keep the steel hard during hot rolling, where the slab is squeezed into a long sheet about two to four millimeters thick. But depending on the metal and how much of it is used, alloying can be costly. “For automotive steels, you want them to be inexpensive,” Dr. Matlock said. “The alloying that goes into them has to be low.”
The next step in transforming the steel relies not on adding metals, but on using temperature to change the arrangement of the atoms in steel. Like most metals, steel has a crystalline structure, with iron and other atoms arranged in orderly fashion. But steel is not a single continuous crystal, like, say, the silicon that is used for semiconductor production. Rather, it consists of crystallites, or grains, that can vary in size and orientation.
To change the crystal structure, manufacturers use techniques like cold rolling, annealing and quenching. The steel sheet is rolled again, at room temperature, and heated to a high temperature for a specific time before being rapidly cooled, or quenched, by water. The process is fast, continuous and precisely controlled.
“Very fast cooling gives us the ability to get unique structures with far less alloying,” Dr. Zuidema said.
The process results in phase transformations, or changes in the steel’s crystalline structure. When the sheet is heated above about 1,300 degrees Fahrenheit, some of the basic cube-shaped crystals, known as ferrite, transform to another cube-shaped phase, austenite. The rapid cooling then converts the austenite to a third phase, martensite, which has an elongated shape and traps more carbon atoms. “Martensite is a very refined structure,” Dr. Matlock said, and adds strength.
The result is a steel with dual phases of ferrite and martensite, and by precisely controlling temperature and timing, the amounts of the two can be varied to produce steels of differing strengths and formability characteristics. Steelmakers can, in effect, dial up the strength and ductility desired. “You get the final structure you want,” Dr. Matlock said, “and create the final properties you need for the specific design.”
The so-called martensitic transformation can be thought of as a slight repositioning of the atoms that reshapes the crystal. The change is so rapid that it travels through the steel at about the speed of sound. Such transformations are not limited to steels, or even metals; the protein crystals in the tail sheath of some viruses, for example, undergo a martensitic transformation, changing their structure rapidly before the tail invades a bacterium.
In some high-strength steels, all of the ferrite ends up being converted to martensite; in others, some austenite remains. High-martensite steels, including those with small amounts of boron added, are difficult to form, so parts are usually made by hot stamping, in which the steel is heated first, stamped and then cooled to allow phase transformation and hardening. Steels with retained austenite are easier to form and get harder during the forming process, or even when crumpling in a crash, as the deformations convert the austenite to martensite.
For the car manufacturer, there is a complex calculus involving strength, weight requirements and costs that go into choosing what steel goes where. A part made of stronger steel may save some weight, but it may require more work to produce the necessary tools and dies. “Tool development costs a lot of money,” said Mr. Schultz, of Ducker Worldwide. Other steels could require different welding methods, so it might not be practical or cost-effective to introduce them into an assembly line.
What is certain, though, is that cars are going to have to continue becoming lighter and stronger. New federal regulations, for example, call for the roof to be able to withstand three times the vehicle’s weight in a rollover, twice the previous standard. And Mr. Schultz has calculated that to meet fuel economy goals for 2020, carmakers will have to keep replacing mild steels, and even some early-generation high-strength grades, with higher-strength steels (and in some cases with aluminum, plastics and other materials).
For steelmakers, that means continuing development of more advanced products that are stronger and yet remain formable. “It’s a systematic engineering process,” said Dr. Zuidema, of ArcelorMittal. “We have a fairly comprehensive knowledge of how each of the potential phases in the iron-carbon system affects the mechanical properties.” So if they know what properties are desired, they can determine the structure needed, and thus the combination of alloying and processing to achieve that structure.
For the steel companies, even incremental improvements will help the car companies meet crash and fuel standards — and help the steelmakers keep other materials manufacturers at bay.
“You’re fighting for ounces here,” Mr. Schultz said. “You’re turning over every rock. I’ve told my steel friends, fasten your seatbelts.”
By HENRY FOUNTAIN
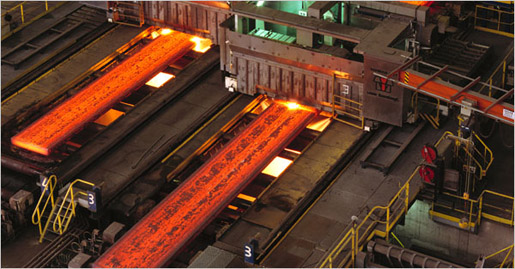
But automotive steel has changed quite a bit since the first Model T rolled off the assembly line. Metallurgists and manufacturers have learned to manipulate steel’s microstructure through precise control of processing to create sheet steels of increasing strength. Prompted by crash-worthiness requirements and the need to make cars lighter to improve gas mileage, automakers are replacing conventional steels with advanced high-strength ones.
Where once a single grade of steel might have sufficed, the typical “body in white,” as automakers call a car’s basic skeleton, might now be a patchwork of a dozen or more steels of different types and strengths, tailored through computer modeling to handle the stress and strain of normal driving — and of severe crashes.
“The day of the mild steel part at the Ford Motor Company is dead,” said Ford’s chief safety engineer, Steve Kozak. “The majority of steels we’re using now are high strength or ultra high strength.”
The advanced steels go by names like D.P. (for dual phase) and TRIP (for transformation-induced plasticity). The strongest ones are used in parts like door beams, where the aim is to stop a foreign object (like another car’s bumper) from entering the passenger compartment, and windshield pillars, where the goal is to prevent the roof from flattening like a pancake in a rollover.
In the front and rear of the vehicle, where there is more room to absorb the energy of a collision, steels that deform more easily, and get stronger as they do, might be used. Even body panels, which are usually made from milder steel, are bake hardenable, getting stronger as they are heated during paint curing to resist denting better.
North American carmakers draw a lot of criticism for overall design and reliability of their cars, but they generally receive high marks for their use of advanced steels. “I’d say North America doesn’t have to take a back seat to anyone,” said Richard A. Schultz of Ducker Worldwide, a consulting firm that tracks materials use by automakers.
The steel industry, Mr. Schultz said, has worked to develop new products, to keep steel in cars and to fend off the increased use of aluminum and other materials. They have been developing higher-strength products for decades, beginning with high-strength low-alloy, or H.S.L.A., steels. But most were costly to produce and difficult to make into parts. “They were looked at as more for aerospace applications,” Mr. Schultz said.
Only in recent years have researchers and manufacturers figured out how to make economical high-strength steels that are pliant enough to be stamped and formed and that can be welded or otherwise joined to other parts in the complex auto assembly process, where time is money.
“At the end of the day we have to manufacture everything that’s there,” said James G. Schroth, lab group manager in the Materials and Processes Lab of General Motors’ Research and Development Center. “The real changes in technology have been the higher-strength materials we can still make into parts.”
Using higher-strength steel, a part can be downgauged — made from thinner stock — to help improve fuel economy. But some parts, notably exterior panels, are already so thin — less than a millimeter, or one twenty-fifth of an inch, in many cases — that stiffness becomes an issue. “You reach a thickness where you can’t go even thinner,” Mr. Schroth said.
Like other steels, high-strength varieties begin as molten iron produced from ore and coke in a blast furnace. “Despite literally hundreds of years of development of the process, it’s still the best and most economical way of making high-purity iron,” said Blake K. Zuidema, director of automotive product applications for the steelmaker ArcelorMittal.
The molten iron, usually with scrap added, is made into steel in a basic oxygen furnace, in which oxygen is blown into the melt (“basic” in the name refers to the alkaline materials used to line the furnace). The gas oxidizes the iron and removes some impurities and most of the carbon. (High-carbon steel is very hard but difficult to work.)
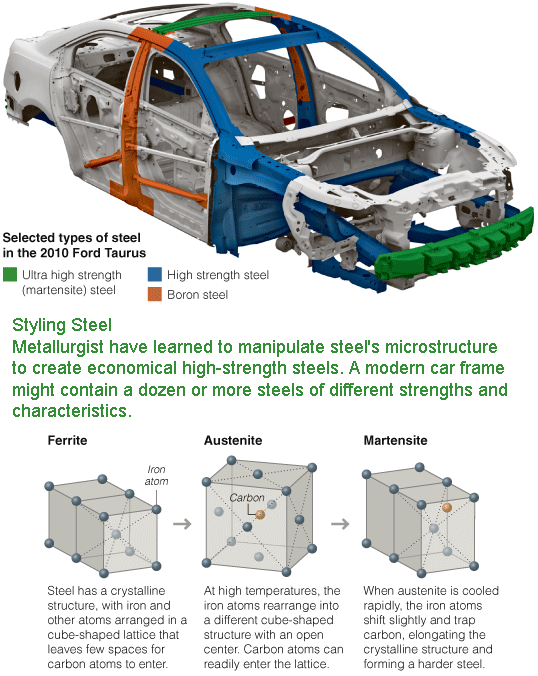
For most automotive purposes, the steel is cast into a slab. At this point, Dr. Zuidema said, “you have a product that is not too much different than conventional steels,” except for the addition of small amounts of other metals — alloys.
Some of the strength of steel is gained through alloying, said David K. Matlock, a metallurgist at the Colorado School of Mines and director of the Advanced Steel Processing and Products Research Center, which is supported by auto and steel companies.
Small amounts of metals like niobium or vanadium help keep the steel hard during hot rolling, where the slab is squeezed into a long sheet about two to four millimeters thick. But depending on the metal and how much of it is used, alloying can be costly. “For automotive steels, you want them to be inexpensive,” Dr. Matlock said. “The alloying that goes into them has to be low.”
The next step in transforming the steel relies not on adding metals, but on using temperature to change the arrangement of the atoms in steel. Like most metals, steel has a crystalline structure, with iron and other atoms arranged in orderly fashion. But steel is not a single continuous crystal, like, say, the silicon that is used for semiconductor production. Rather, it consists of crystallites, or grains, that can vary in size and orientation.
To change the crystal structure, manufacturers use techniques like cold rolling, annealing and quenching. The steel sheet is rolled again, at room temperature, and heated to a high temperature for a specific time before being rapidly cooled, or quenched, by water. The process is fast, continuous and precisely controlled.
“Very fast cooling gives us the ability to get unique structures with far less alloying,” Dr. Zuidema said.
The process results in phase transformations, or changes in the steel’s crystalline structure. When the sheet is heated above about 1,300 degrees Fahrenheit, some of the basic cube-shaped crystals, known as ferrite, transform to another cube-shaped phase, austenite. The rapid cooling then converts the austenite to a third phase, martensite, which has an elongated shape and traps more carbon atoms. “Martensite is a very refined structure,” Dr. Matlock said, and adds strength.
The result is a steel with dual phases of ferrite and martensite, and by precisely controlling temperature and timing, the amounts of the two can be varied to produce steels of differing strengths and formability characteristics. Steelmakers can, in effect, dial up the strength and ductility desired. “You get the final structure you want,” Dr. Matlock said, “and create the final properties you need for the specific design.”
The so-called martensitic transformation can be thought of as a slight repositioning of the atoms that reshapes the crystal. The change is so rapid that it travels through the steel at about the speed of sound. Such transformations are not limited to steels, or even metals; the protein crystals in the tail sheath of some viruses, for example, undergo a martensitic transformation, changing their structure rapidly before the tail invades a bacterium.
In some high-strength steels, all of the ferrite ends up being converted to martensite; in others, some austenite remains. High-martensite steels, including those with small amounts of boron added, are difficult to form, so parts are usually made by hot stamping, in which the steel is heated first, stamped and then cooled to allow phase transformation and hardening. Steels with retained austenite are easier to form and get harder during the forming process, or even when crumpling in a crash, as the deformations convert the austenite to martensite.
For the car manufacturer, there is a complex calculus involving strength, weight requirements and costs that go into choosing what steel goes where. A part made of stronger steel may save some weight, but it may require more work to produce the necessary tools and dies. “Tool development costs a lot of money,” said Mr. Schultz, of Ducker Worldwide. Other steels could require different welding methods, so it might not be practical or cost-effective to introduce them into an assembly line.
What is certain, though, is that cars are going to have to continue becoming lighter and stronger. New federal regulations, for example, call for the roof to be able to withstand three times the vehicle’s weight in a rollover, twice the previous standard. And Mr. Schultz has calculated that to meet fuel economy goals for 2020, carmakers will have to keep replacing mild steels, and even some early-generation high-strength grades, with higher-strength steels (and in some cases with aluminum, plastics and other materials).
For steelmakers, that means continuing development of more advanced products that are stronger and yet remain formable. “It’s a systematic engineering process,” said Dr. Zuidema, of ArcelorMittal. “We have a fairly comprehensive knowledge of how each of the potential phases in the iron-carbon system affects the mechanical properties.” So if they know what properties are desired, they can determine the structure needed, and thus the combination of alloying and processing to achieve that structure.
For the steel companies, even incremental improvements will help the car companies meet crash and fuel standards — and help the steelmakers keep other materials manufacturers at bay.
“You’re fighting for ounces here,” Mr. Schultz said. “You’re turning over every rock. I’ve told my steel friends, fasten your seatbelts.”
By HENRY FOUNTAIN
You can return to the main Market News page, or press the Back button on your browser.