Chemical industry sees green
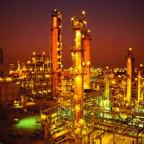
“We have made tremendous strides over the past decade-and-a-half in reversing the psychology of industry and government on the matter of pollution prevention,” says Paul Anastas, “and yet we have barely scratched the surface of what the promise of green chemistry holds.” Indeed, beneath that surface may lie the future for whole swathes of the chemical industry, as the drive to “go green” grows more persuasive and pervasive.
As director of the Green Chemistry Institute of the American Chemical Society, Washington, D.C., and former assistant director for the environment in the White House Office of Science and Technology Policy, Anastas has been one of the champions of green technology and was one of the prime movers behind the 1997 establishment of the U.S. Environmental Protection Agency’s Presidential Green Chemistry Challenge Awards. Moving on this month to head up the new Center for Green Chemistry and Green Engineering at Yale University, New Haven, Conn., he has defined green chemistry as “the design of new products and processes that reduce or eliminate the use and generation of hazardous substances … we’re really talking about the chemistry of sustainability.”
But if pollution prevention was the original goal of green chemistry, the efforts of Anastas and researchers like him have seen the philosophy develop into one that promises as much economic as environmental benefit. The 2006 Presidential Award winners, for example, include Galen Suppes, professor of chemical engineering at the University of Missouri - Columbia, whose catalytic process for converting glycerol (glycerin) into propylene glycol could change the economics of the burgeoning biodiesel industry.
Byproduct upgrade
Biodiesel economics strongly depend on the market for glycerol, biodiesel’s byproduct from the transesterification of vegetable oils. The U.S. biodiesel industry is expected to introduce 1 billion pounds of additional glycerol into a market that currently only has an annual demand for 600 million pounds. So, the industry clearly needs to find a high-value use for its glycerol. Propylene glycol (PG), a less toxic alternative to ethylene glycol for antifreeze and many other uses, could be the answer. Producing it from glycerol, says Suppes, can reduce the cost of biodiesel manufacture by as much as $0.40/gal.
Suppes’ award-winning process couples a new copper-chromite catalyst with reactive distillation and offers a number of advantages, such as lower operating temperature and pressure, more efficient conversion and less byproduct, compared to previous conversion routes. The same technology also can be used to convert glycerol to acetol or hydroxyacetone, an intermediate and monomer used in the production of polyols. When made from petroleum, acetol costs around $5/lb, which limits its widespread use. However, using biomass-sourced glycerol could cut production cost to as little as $0.50/lb, opening up more markets for glycerol and so benefiting biodiesel production.
Other researchers also are investigating ways of catalytically upgrading glycerol to PG. Under the auspices of the Office of Energy Efficiency and Renewable Energy of the U.S. Department of Energy (DOE), Washington, D.C., researchers at Michigan State University, East Lansing, Mich., and the Pacific Northwest National Laboratory (PNNL), Richland, Wash., are collaborating with Archer Daniels Midland, Decatur, Ill., and UOP, Des Plaines, Ill., on the development of catalysts for an integrated process. A team led by John Holladay at PNNL has used high-throughput combinatorial techniques to screen more than 4,000 possible catalysts. (For more on developments in high-throughput methods, see R&D takes the fast track.) The next stages in the program, which runs through 2008, involve reaction evaluation and pilot scale testing and finally a conceptual design for a commercial unit.
Meanwhile, food and agricultural giant Cargill, Minneapolis, Minn., is forming a new company to make PG from renewable feedstocks using a proprietary process that is said to increase production efficiency by providing better yields and fewer byproducts than other renewable and non-renewable routes. “Cargill already sells glycerin from its biodiesel plants and has ready access through its supply chain and other sources to produce enough glycerin for world-scale production of PG,” says Jim Stoppert, Cargill’s senior director of industrial bioproducts. “We are confident that our approach to manufacturing price-competitive, renewable PG on a large commercial scale will be highly desired by the chemical industry.” Initial indications are that the product won’t require reformulation prior to downstream use. Cargill expects “market rollout to occur quickly.”
Bioethanol boom
Despite the boost that such developments might give to the biodiesel industry, the biggest impact of green fuel technology will almost certainly come from bioethanol as a gasoline replacement. Current U.S. production totals around 4 billion gal/yr, mostly from corn, but there is rapidly growing interest in developing biorefineries able to process a range of feedstocks including non-foodstuff renewable biomass materials.
With biotechnology processes currently available that use enzymes to convert biomass to fermentable sugars, the U.S. could produce more than 70 billion gallons of cellulosic ethanol (as opposed to the conventional grain-derived bioethanols) per year from crop residues such as corn stover and stalks, sugar cane bagasse, wheat straw and rice straw, according to the Biotechnology Industry Organization, Washington, D.C. Jim Greenwood, the organization’s president and CEO, says 25% of the nation’s transportation fuel could be supplied from biorefineries by 2015 if development were dramatically ramped up.
It’s still very much a fledging industry but the first seeds have already taken root around the world. A demonstration plant in Ottawa, Ont., started up in 2004 by Iogen of Ottawa, provided the first pre-commercial-scale output of cellulosic ethanol. Designed to produce up to 3 million liters/yr, the plant can handle up to 40 metric tons/d of raw material feedstock such as wheat, oat and barley straw, enzymatically converting the cellulose fiber into glucose for fermentation to ethanol.
The first true commercial-scale plant, however, was scheduled for start-up in December in Babilfuente, Spain, at the BCyl cereal ethanol plant of Abengoa Bioenergy, Seville, Spain, and St Louis, Mo. The ethanol-from-biomass plant, which has a 5-million-liter/yr capacity from a feed of 70 metric tons/d of agricultural residue, has been constructed alongside a far larger (195-million-liter/yr) cereal ethanol plant to benefit from its infrastructure. Abengoa, the world’s second largest bioethanol producer, is utilizing some of the latest technologies in the new plant, including a continuous biomass pretreatment system from SunOpta, Toronto, Ont., and new enzymes from Novozymes A/S, Bagsvaerd, Denmark, and Franklinton, N.C., developed in conjunction with the DOE’s National Renewable Energy Laboratory, Golden, Colo.
In June 2006, both SunOpta and Novozymes signed a contract with China Resource Alcohol Corp. (CRAC) for a cellulosic-ethanol demonstration plant in ZhaoDong City, China. CRAC’s goal is to install 1.7-million-gal/yr capacity by the end of this year and 330 million gal/yr by 2012.
Another major cellulosic-ethanol project in the works is a 30-million-liter/yr unit in Orange County, Calif., that will use process technology from Arkenol, Irvine, Calif. The plant, being engineered by JGC Corp., Yokohama, Japan, and Houston, Texas, is due for start-up early in 2009.
Going beyond fuels
For biorefineries to fulfill their promise, however, downstream markets other than those for fuels also need attention. “It seems unlikely that fuel from a biorefinery — at least in the beginning — is going to be as cost-effective as fuel from traditional fossil sources. To make the biorefinery sustainable, we must therefore do everything we can to help the economics,” says Charles Eckert, a professor in the school of chemical and biomolecular engineering at the Georgia Institute of Technology, Atlanta, Ga., and another Presidential Green Chemistry Challenge award winner.
Eckert and a colleague, Charles Liotta, won the 2004 Presidential award for their development of benign tunable solvents that couple reaction and separation processes. They are now collaborating with other researchers on the potential for producing high-value (up to $25/lb) specialty chemicals, pharmaceutical precursors and flavorings as “side stream” chemicals from the ethanol process (Figure 1).
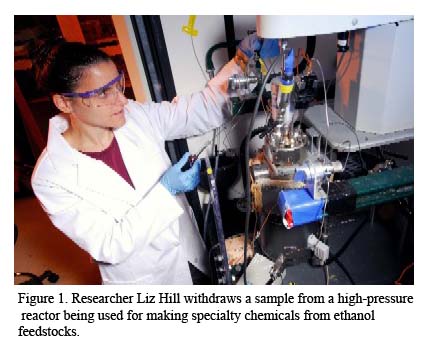
“These are novel feedstocks for chemical production,” Eckert notes. “They are very different from what we’ve dealt with before. This gives us different challenges and provides a rich area for interdisciplinary research.” Working as part of a research alliance with Oak Ridge National Laboratory, Oak Ridge, Tenn., and Imperial College, London, U.K., the team is exploring solvent techniques such as the use of near-critical water (pressurized water at 250°C to 300°C), gas-expanded liquids (such as CO2 in methanol) and supercritical fluids (CO2 under high pressure).
Meanwhile at the reaction level, Isis Innovation, Oxford, U.K., a company that promotes commercialization of developments at Oxford University, has licensed its Cytochrome P-450 designer enzyme technology to Industrial Biotechnology Corp. (IBC), Sarasota, Fla. Developed at the university by chemistry professor Luet Wong, the P-450 technology applies specific enzymes as biocatalysts for the conversion, often in a single step, of relatively low value substrates into high value chemicals. IBC says the potential market embraces more than 15,000 commercially available chemicals, including various alcohols, aldehydes, ketones and carboxylic acids.
Plastics’ progress
The production of plastics from renewable resources provides another example of how green technologies could change the face of the chemical industry. In 2006, for example, Archer Daniels Midland announced plans to build the first commercial plant to produce PHAs (polyhydroxylalkanoates), a family of biodegradable high-performance plastics that can be used in many applications currently served by petrochemical plastics — e.g., coatings, film and molded goods. Due for completion by the middle of 2008, the 50,000-ton/yr plant at Clinton, Iowa, will serve the joint venture set up between ADM and Metabolix, Cambridge, Mass., the company that developed the proprietary PHA technology and won a Presidential Award in 2004 for its work on commercializing bioplastics through the use of metabolic engineering and molecular biology.
Commenting on the joint venture, Jim Barber, Metabolix president and CEO, says “a broadly useful family of bio-based, biodegradable natural plastics will be commercially available for the first time.” Located next to ADM’s existing wet corn mill at Clinton, the PHA plant will take starch from the mill as its raw material.
Cargill subsidiary NatureWorks, Minnetonka, Minn., already offers a biodegradable plastic. Its polylactic acid (PLA) polymer is produced from corn at a 140,000-metric-ton/yr plant in Blair, Neb. PLA already has won a significant place in biodegradable packaging; Wal-Mart switched to PLA packaging for its fruit and herbs in 2005.
Not content with producing an inherently biodegradable product from a renewable feedstock, however, the NatureWorks plant has taken the application of green technology even further. Through the purchase of renewable energy certificates it has effectively replaced all the fossil-based electricity used in the plant with wind power. And, according to the company, by buying additional certificates to offset remaining greenhouse gas emissions, its PLA will be the world’s first and only greenhouse-gas-neutral polymer. Strictly speaking, PLA polymers fall more within the definition of compostable rather than biodegradable materials at least in the U.S. (The difference is one of timescale and the ambient conditions required for each.) They can, however, also be recycled, which for the moment is arguably one of the few ways for petrochemical-based plastics to carry a “green” label — a case in point being the new use for recycled polyethylene terephthalate (PET) waste developed by GE Plastics, Pittsfield, Mass.
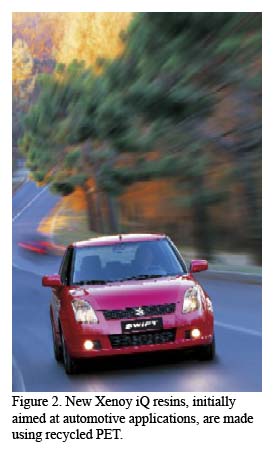
Plastic-waste feedstock
GE has developed a new route to polybutylene terephthalate (PBT) based resins and polyester-based elastomers that uses PET waste — mainly from plastic bottles — as most of its feedstock. According to Vikram Gopal, GE Plastics’ program manager for crystalline plastics, the PET is first depolymerized and then chemically upgraded so it can be reacted with butanediol (BDO) — one of the main feedstocks in the conventional process — to produce PBT. The recycled-waste-based material supplants the other conventional feedstocks, either dimethyl terephthalate (DMT) or terephthalic acid (TPA). In all, more than 85% of PBT’s usual feedstocks are replaced by this “post-consumer feedstock.” The Valox iQ and Xenoy iQ resins made from the new PBT-based polymers are initially targeted at automotive applications (Figure 2).
GE says that if all the PBT produced in 2005 had been in the form of Valox iQ and Xenoy iQ, it would have consumed more than 565,000 metric tons of PET waste, the equivalent of 22.5 billion plastic bottles. While those are certainly impressive “green” credentials, the figures also help to put some perspective on the scale of the green chemical industry as it currently stands. For example, the annual global production capacity for biodegradable materials amounts to only around 300,000 metric tons, with the NatureWorks plant accounting for almost half of that, according to industry association European Bioplastics, Berlin, Germany.
With retail giants like Wal-Mart already in the market for biodegradable packaging, this level of capacity undoubtedly only scratches the surface of the potential for this particular green chemistry.
On a wider front, the chemical industry clearly is starting to unwrap the potential benefits of “going green.”
For more information please visit, Chemical Processing.
You can return to the main Market News page, or press the Back button on your browser.